Jan
12
One Step Closer To Economical Water Splitting For Hydrogen
January 12, 2021 | Leave a Comment
Efficiently mass-producing hydrogen from water is closer to becoming a reality thanks to new findings. The research came from Oregon State University College of Engineering researchers and collaborators at Cornell University and the Argonne National Laboratory.
The scientists used advanced experimental tools to forge a clearer understanding of an electrochemical catalytic process that’s cleaner and more sustainable than deriving hydrogen from natural gas.
The group’s findings have been published in Science Advances.
Hydrogen is found in a wide range of compounds on Earth, most commonly combining with oxygen to make water, and it has many scientific, industrial and energy-related roles. It also occurs in the form of hydrocarbons, compounds consisting of hydrogen and carbon such as methane, the primary component of natural gas.
Oregon State’s Zhenxing Feng, a chemical engineering professor who led the study said, “The production of hydrogen is important for many aspects of our life, such as fuel cells for cars and the manufacture of many useful chemicals such as ammonia. It’s also used in the refining of metals, for producing human-made materials such as plastics and for a range of other purposes.”
The data at the U.S. Department of Energy shows the United States produces most of its hydrogen from a methane source such as natural gas via a technique known as steam-methane reforming. The process involves subjecting methane to pressurized steam in the presence of a catalyst, creating a reaction that produces hydrogen and carbon monoxide, as well as a small amount of carbon dioxide.
The next step is referred to as the water-gas shift reaction in which the carbon monoxide and steam are reacted via a different catalyst, making carbon dioxide and additional hydrogen. In the last step, pressure-swing adsorption, carbon dioxide and other impurities are removed, leaving behind pure hydrogen.
Feng explained, “Compared to natural gas reforming, the use of electricity from renewable sources to split water for hydrogen is cleaner and more sustainable. However, the efficiency of water-splitting is low, mainly due to the high overpotential – the difference between the actual potential and the theoretical potential of an electrochemical reaction – of one key half-reaction in the process, the oxygen evolution reaction or OER.”
A half-reaction is either of the two parts of a redox, or reduction-oxidation, reaction in which electrons are transferred between two reactants; reduction refers to gaining electrons, oxidation means losing electrons.
The concept of half-reactions is often used to describe what goes on in an electrochemical cell, and half-reactions are commonly used as a way to balance redox reactions. Overpotential is the margin between the theoretical voltage and the actual voltage necessary to cause electrolysis – a chemical reaction driven by the application of electric current.
Feng, in outlining the basics said, “Electrocatalysts are critical to promoting the water-splitting reaction by lowering the overpotential, but developing high-performance electrocatalysts is far from straightforward. One of the major hurdles is the lack of information regarding the evolving structure of the electrocatalysts during the electrochemical operations. Understanding the structural and chemical evolution of the electrocatalyst during the OER is essential to developing high-quality electrocatalyst materials and, in turn, energy sustainability.”
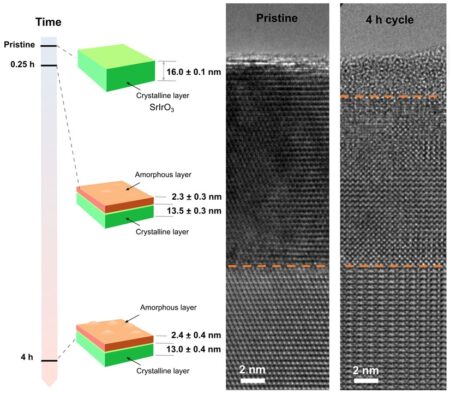
(Left) The formed amorphous IrOx layer appears to reach a steady-state thickness by 0.25 hours. (Right) Cross-section transmission electron microscopy (TEM) images of the pristine SrIrO3 film after 4 hours of potential cycling between 1.05 and 1.75 V versus RHE.
Feng and the collaborators used a set of advanced characterization tools to study the atomic structural evolution of a state-of-the art OER electrocatalyst, strontium iridate (SrIrO3), in acid electrolyte.
“We wanted to understand the origin of its record-high activity for the OER – 1,000 times higher than the common commercial catalyst, iridium oxide,” Feng said. “Using synchrotron-based X-ray facilities at Argonne and lab-based X-ray photoelectron spectroscopy at the Northwest Nanotechnology Infrastructure site at OSU, we observed the surface chemical and crystalline-to-amorphous transformation of SrIrO3 during the OER.”
The observations led to a deep understanding of what was going on behind strontium iridate’s ability to work so well as a catalyst.
Feng summed up, “Our detailed, atomic-scale finding explains how the active strontium iridate layer forms on strontium iridate and points to the critical role of the lattice oxygen activation and coupled ionic diffusion on the formation of the active OER units.”
Feng added that the work provides insight into how applied potential facilitates the formation of the functional amorphous layers at the electrochemical interface and leads to possibilities for the design of better catalysts.
This post comes from one of the best press releases seen in quite some time. Your humble writer thanks Steve Lundeberg for both the research information as well as a brief and yet highly instructive review of current hydrogen production and the issues faced in getting to economical water splitting.
This research isn’t a slam dunk solution to the water splitting dilemma. But it sure does illuminate the research in catalysts such that more progress can be made. For that – it might well be a breakthrough.