May
21
Osaka Metropolitan University researchers have found that persimmon tannin, known for its antioxidant properties, improves the growth of yeast in the presence of ethanol. It’s a case of naturally derived antioxidants improving growth of yeast strain in presence of ethanol.
The findings have been published in the Journal of the Science of Food and Agriculture.
While ethanol in alcoholic beverages impairs drinkers’ motor functions, it is that same substance that can power motor vehicles in a cleaner, more sustainable manner. What is necessary for the production of ethanol is yeast, but ethanol is among the environmental factors that add stress to yeasts, hindering their growth. To promote efficient bioethanol production, scientists have been searching for substances that can help yeasts better withstand ethanol, but few effective ones have been found.
An Osaka Metropolitan University research team, including graduate student Ilhamzah and Professor Ken-ichi Fujita of the Graduate School of Science and Professor Akira Ogita of the Research Center for Urban Health and Sports, has found that tannin from persimmons improves the growth of the yeast strain Saccharomyces cerevisiae in the presence of ethanol.
Professor Fujita stated, “In this study, yeast cultures grown in a medium containing ethanol and persimmon tannin showed an 8.9-fold increase in cell number compared to cultures grown in an ethanol medium without persimmon tannin.”
The researchers explored persimmon tannin because it is known for its antioxidative properties.
“Persimmon tannin reduced ethanol-induced oxidative stress,” Fujita added.
“However, persimmon tannin did not prevent ethanol-induced cell membrane damage. This indicates the potential of persimmon tannin as a protective agent to enhance the yeast’s tolerance to ethanol stress by limiting oxidative damage, rather than limiting damage to the yeast’s cell membranes.”
**
Persimmon isn’t likely in many folks hot list of flavors. But the breadth and depth of the research is what surprises.
It may well be a part of a solution that increases ethanol production efficiency and that is always a welcome thing.
May
16
EPFL (École Polytechnique Fédérale de Lausanne) researchers have used a genetic learning algorithm to identify optimal pitch profiles for the blades of vertical-axis wind turbines. Vertical-axis wind turbines with their high energy potential, have until now been vulnerable to strong gusts of wind.
The explanatory open access paper has been published Nature Communications.
When you consider today’s industrial wind turbine, you likely picture the windmill design, technically known as a horizontal-axis wind turbine (HAWT). But the very first wind turbines, which were developed in the Middle East around the 8th century for grinding grain, were vertical-axis wind turbines (VAWT), meaning they spun perpendicular to the wind, rather than parallel.
Due to their slower rotation speed, VAWTs are less noisy than HAWTs and achieve greater wind energy density, meaning they need less space for the same output both on- and off-shore. The blades are also more wildlife-friendly: because they rotate laterally, rather than slicing down from above, they are easier for birds to avoid.
With these advantages, why are VAWTs largely absent from today’s wind energy market? As Sébastien Le Fouest, a researcher in the School of Engineering Unsteady Flow Diagnostics Lab (UNFOLD) explains, it comes down to an engineering problem – air flow control – that he believes can be solved with a combination of sensor technology and machine learning. In the paper recently published in Nature Communications, Le Fouest and UNFOLD head Karen Mulleners describe two optimal pitch profiles for VAWT blades, which achieve a 200% increase in turbine efficiency and a 77% reduction in structure-threatening vibrations.

EPFL’s experimental VAWT blade Image Credit: © UNFOLD EPFL CC BY SA. Click the press release link for more and larger images.
Le Fouest noted, “Our study represents, to the best of our knowledge, the first experimental application of a genetic learning algorithm to determine the best pitch for a VAWT blade.”
Turning an Achilles’ heel into an advantage
Le Fouest explained that while Europe’s installed wind energy capacity is growing by 19 gigawatts per year, this figure needs to be closer to 30 GW to meet the UN’s 2050 objectives for carbon emissions.
“The barriers to achieving this are not financial, but social and legislative – there is very low public acceptance of wind turbines because of their size and noisiness,” he said.
Despite their advantages in this regard, VAWTs suffer from a serious drawback: they only function well with moderate, continuous air flow. The vertical axis of rotation means that the blades are constantly changing orientation with respect to the wind. A strong gust increases the angle between air flow and blade, forming a vortex in a phenomenon called dynamic stall. These vortices create transient structural loads that the blades cannot withstand.
To tackle this lack of resistance to gusts, the researchers mounted sensors onto an actuating blade shaft to measure the air forces acting on it. By pitching the blade back and forth at different angles, speeds, and amplitudes, they generated series of ‘pitch profiles’. Then, they used a computer to run a genetic algorithm, which performed over 3500 experimental iterations. Like an evolutionary process, the algorithm selected for the most efficient and robust pitch profiles, and recombined their traits to generate new and improved ‘offspring’.
This approach allowed the researchers not only to identify two pitch profile series that contribute to significantly enhanced turbine efficiency and robustness, but also to turn the biggest weakness of VAWTs into a strength.
“Dynamic stall – the same phenomenon that destroys wind turbines – at a smaller scale can actually propel the blade forward. Here, we really use dynamic stall to our advantage by redirecting the blade pitch forward to produce power,” Le Fouest explained. “Most wind turbines angle the force generated by the blades upwards, which does not help the rotation. Changing that angle not only forms a smaller vortex – it simultaneously pushes it away at precisely the right time, which results in a second region of power production downwind.”
The Nature Communications paper represents Le Fouest’s PhD work in the UNFOLD lab. Now, he has received a BRIDGE grant from the Swiss National Science Foundation (SNSF) and Innosuisse to build a proof-of-concept VAWT. The goal is to install it outdoors, so that it can be tested as it responds in real time to real-world conditions.
“We hope this air flow control method can bring efficient and reliable VAWT technology to maturity so that it can finally be made commercially available,” Le Fouest said.
**
One does certainly hope this development has the wherewithal to replace a lot of those dangerous ugly and noisy HAWTs. While wind is a notorious intermittent power source the industry has a lot of momentum that sucks up immense amounts of ratepayer and taxpayer money. Stamping out rent seeking plans like wind turbines could serve as an example of how terribly an economy and its citizens are damaged by political enforced rent making schemes.
It would be great of the developers could say the technology can stand economically on its own. But the press release makes no such comments. The reality is these can only supplement a bit when the winds blows.
May
15
Boosting Solar Energy Efficacy In Solar Panels
May 15, 2024 | Leave a Comment
The researchers found that by integrating these reflectors into solar setups, they could improve the system’s energy production and efficiency, making such projects more economically viable. This discovery is significant in assessing the costs and benefits of using artificial reflectors in solar energy ventures.
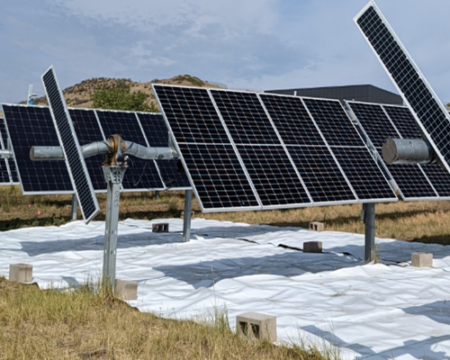
Test site photo of reflective underlayment for solar panels of an artificial reflector experiment performed on the Bifacial Experimental Single-Axis-Tracking Site (BEST Site) at NREL in Golden, Colorado. Image Credit: National Renewable Energy Laboratory, Golden Colorado. For more and larger images click the press release link here.
To study how reflective ground covers affect solar energy output, the University of Ottawa’s SUNLAB, led by electrical engineering Professor Karin Hinzer, who is also vice-dean, research of the Faculty of Engineering, collaborated with the National Renewable Energy Laboratory (NREL) in Golden, Colorado, a world leader in clean energy research, development, and deployment. The study, which was conducted by electrical engineering doctoral candidate Mandy Lewis in Golden, Colorado, found that placing reflective surfaces under solar panels can increase their energy output by up to 4.5%.
“We found that highly reflective white surfaces can boost solar power output,” explains Mandy Lewis, the paper’s lead author. “Critically, these reflectors should be placed directly under the solar panels, not between rows, to maximize this benefit.”
Unlocking solar potential in Canada and beyond
These findings are particularly significant in Canada, where snow cover persists for three-to four months of the year in major cities like Ottawa and Toronto, and 65% of the country’s vast landmass experiences snow cover for over half the year. Bifacial solar systems, paired with high ground reflectivity, offer tremendous potential in these regions. Additionally, given that approximately 4% of the world’s land areas are classified as sandy deserts, this finding has global applications.
According to Lewis, “this research is crucial for maximizing solar energy production in geographically diverse locations. Furthermore, by generating more power per unit of land area, reflectors are ideal for densely populated areas, like city centres, where space limitations exist for solar installations.”
This study marks the beginning of a new international research collaboration between the University of Ottawa and NREL. The project was funded by the National Sciences and Engineering Research Council of Canada (NSERC), Ontario Graduate Scholarships (OGS), and the US Department of Energy (DoE), underscoring the importance of collaborative efforts in advancing renewable energy technologies.
Global impact in facilitating the transition to clean energy
This research will contribute significantly to the global transition to zero-emission power sources. These findings hold particular value for Canada and other countries that are typically cloudy, since power gains of 6.0% were observed in cloudy Seattle compared to 2.6% in arid Tucson.
**
The photos suggest this technology would be applicable across quite a range of locations. It also looks like there are solar cell panels facing down from under the panel. Just how this technology might cost out isn’t discussed, but for high latitudes and climates with lots of cloudy time compared to clear weather this might be a workable solution.
On the other hand, solar at high latitude or in cloudy locations has a very difficult cost barrier to overcome. While this technology is inventive and innovative, the price to get into service is worrisome, both as an expense, maintenance and as a subject for government policy forcing the costs on consumers.
May
14
In a just released Northwestern University study, the new catalyst successfully converted CO2 into CO, an important building block to produce a variety of useful chemicals. When the reaction occurs in the presence of hydrogen, for example, CO2 and hydrogen transform into synthesis gas (or syngas), a highly valuable precursor to producing fuels that can potentially replace gasoline.

Schematic Image Credit:Milad Khoshooei at Northwestern University. Click here for the press release page.
With recent advances in carbon capture technologies, post-combustion carbon capture is becoming a plausible option to help tackle the global climate change crisis. But how to handle the captured carbon remains an open-ended question. The new catalyst potentially could provide one solution for disposing the potent greenhouse gas by converting it into a more valuable product.
“Even if we stopped emitting CO2 now, our atmosphere would still have a surplus of CO2 as a result of industrial activities from the past centuries,” said Northwestern’s Milad Khoshooei, who co-led the study. “There is no single solution to this problem. We need to reduce CO2 emissions and find new ways to decrease the CO2 concentration that is already in the atmosphere. We should take advantage of all possible solutions.”
“We’re not the first research group to convert CO2 into another product,” said Northwestern’s Omar K. Farha, the study’s senior author. “However, for the process to be truly practical, it necessitates a catalyst that fulfills several crucial criteria: affordability, stability, ease of production and scalability. Balancing these four elements is key. Fortunately, our material excels in meeting these requirements.”
An expert in carbon capture technologies, Farha is the Charles E. and Emma H. Morrison Professor of Chemistry at Northwestern’s Weinberg College of Arts and Sciences. After starting this work as a Ph.D. candidate at the University of Calgary in Canada, Khoshooei now is a postdoctoral fellow in Farha’s laboratory.
Solutions from the pantry
The secret behind the new catalyst is molybdenum carbide, an extremely hard ceramic material. Unlike many other catalysts that require expensive metals, such as platinum or palladium, molybdenum is an inexpensive, non-precious, Earth-abundant metal.
To transform molybdenum into molybdenum carbide, the scientists needed a source of carbon. They discovered a cheap option in an unexpected place: the pantry. Surprisingly, sugar — the white, granulated kind found in nearly every household — served as an inexpensive, convenient source of carbon atoms.
“Every day that I tried to synthesize these materials, I would bring sugar to the lab from my home,” Khoshooei said. “When compared to other classes of materials commonly used for catalysts, ours is incredibly inexpensive.”
Successfully selective and stable
When testing the catalyst, Farha, Khoshooei and their collaborators were impressed by its success. Operating at ambient pressures and high temperatures (300-600° C), the catalyst converted CO2 into CO with 100% selectivity.
High selectivity means that the catalyst acted only on the CO2 without disrupting surrounding materials. In other words, industry could apply the catalyst to large volumes of captured gases and selectively target only the CO2. The catalyst also remained stable over time, meaning that it stayed active and did not degrade.
“In chemistry, it’s not uncommon for a catalyst to lose its selectivity after a few hours,” Farha said. “But, after 500 hours in harsh conditions, its selectivity did not change.”
This is particularly remarkable because CO2 is a stable — and stubborn — molecule.
“Converting CO2 is not easy,” Khoshooei said. “CO2 is a chemically stable molecule, and we had to overcome that stability, which takes a lot of energy.
Tandem approach to carbon clean-up
Developing materials for carbon capture is a major focus of Farha’s laboratory. His group develops metal-organic frameworks (MOFs), a class of highly porous, nano-sized materials that Farha likens to “sophisticated and programmable bath sponges.” Farha explores MOFs for diverse applications, including pulling CO2 directly from the air.
Now, Farha says MOFs and the new catalyst could work together to play a role in carbon capture and sequestration.
“At some point, we could employ a MOF to capture CO2, followed by a catalyst converting it into something more beneficial,” Farha suggested. “A tandem system utilizing two distinct materials for two sequential steps could be the way forward.”
“This could help us answer the question: ‘What do we do with captured CO2?’” Khoshooei added. “Right now, the plan is to sequester it underground. But underground reservoirs must meet many requirements in order to safely and permanently store CO2. We wanted to design a more universal solution that can be used anywhere while adding economic value.”
Farha is a member of the International Institute for Nanotechology and a faculty affiliate of the Paula M. Trienens Institute for Sustainability and Energy.
**
This catalyst technology just might be what is needed to get CO2 recycling going. It’s such a pity that there is so much global warming scam involved in the press release. One tolerates it as getting further into the recycling research is going to need funding and for now the warming folks have their mitts on the wallet.
For now, the biologists, agronomists and botanists are horrified by this kind of reporting. Cleaning up CO2 makes knowledgeable folks wonder where the food for creatures is going to come from if humans “clean up” the food source away from green plants. What is it that cherry orange and apple trees, wheat, rice, corn, potatoes, poplar, algae, miscanthus, and sugar cane consume to live and grow? CO2
May
9
Lawrence Berkeley National Laboratory scientists have developed microcapacitors with ultrahigh energy and power density, paving the way for on-chip energy storage in electronic devices. Many readers have seen the populations of capacitors installed to computer motherboards and other power intensive silicon chip circuit boards.
The findings, published in the journal Nature, pave the way for advanced on-chip energy storage and power delivery in next-generation electronics.
In the ongoing quest to make electronic devices ever smaller and more energy efficient, researchers want to bring energy storage directly onto microchips, reducing the losses incurred when power is transported between various device components. To be effective, on-chip energy storage must be able to store a large amount of energy in a very small space and deliver it quickly when needed – requirements that can’t be met with existing technologies.
Addressing this challenge, scientists at Lawrence Berkeley National Laboratory (Berkeley Lab) and UC Berkeley have achieved record-high energy and power densities in microcapacitors made with engineered thin films of hafnium oxide and zirconium oxide, using materials and fabrication techniques already widespread in chip manufacturing.

Microcapacitors made with engineered hafnium oxide/zirconium oxide films in 3D trench capacitor structures – the same structures used in modern microelectronics – achieve record-high energy storage and power density, paving the way for on-chip energy storage. Image Credit: Nirmaan Shanker/Suraj Cheema, Lawrence Berkeley National Laboratory. Click the press release link for a larger and more images.
Sayeef Salahuddin, the Berkeley Lab faculty senior scientist and UC Berkeley professor who led the project explained, “We’ve shown that it’s possible to store a lot of energy in microcapacitors made from engineered thin films, much more than what is possible with ordinary dielectrics. What’s more, we’re doing this with a material that can be processed directly on top of microprocessors.”
This research is part of broader efforts at Berkeley Lab to develop new materials and techniques for smaller, faster, and more energy-efficient microelectronics.
Capacitors are one of the basic components of electrical circuits but they can also be used to store energy. Unlike batteries, which store energy through electrochemical reactions, capacitors store energy in an electric field established between two metallic plates separated by a dielectric material. Capacitors can be discharged very rapidly when needed, allowing them to deliver power quickly, and they do not degrade with repeated charge-discharge cycles, giving them much longer lifespans than batteries. However, capacitors generally have much lower energy densities than batteries, meaning they can store less energy per unit volume or weight, and that problem only gets worse when you try to shrink them down to microcapacitor size for on-chip energy storage.
At Lawrence Berkeley National Lab the researchers achieved their record-breaking microcapacitors by carefully engineering thin films of HfO2-ZrO2 to achieve a negative capacitance effect. Normally, layering one dielectric material on top of another results in an overall lower capacitance. However, if one of those layers is a negative-capacitance material, then the overall capacitance actually increases. In earlier work, Salahuddin and colleagues demonstrated the use of negative capacitance materials to produce transistors that can be operated at substantially lower voltages than conventional MOSFET transistors. Here, they harnessed negative capacitance to produce capacitors capable of storing greater amounts of charge, and therefore energy.
The crystalline films are made from a mix of HfO2 and ZrO2 grown by atomic layer deposition, using standard materials and techniques from industrial chip fabrication. Depending on the ratio of the two components, the films can be ferroelectric, where the crystal structure has a built-in electric polarization, or antiferroelectric, where the structure can be nudged into a polar state by applying an electric field. When the composition is tuned just right, the electric field created by charging the capacitor balances the films at the tipping point between ferroelectric and antiferroelectric order, and this instability gives rise to the negative capacitance effect where the material can be very easily polarized by even a small electric field.
Suraj Cheema, a postdoc in Salahuddin’s group and one of the lead authors of the paper explained, “That unit cell really wants to be polarized during the phase transition, which helps produce extra charge in response to an electric field. This phenomenon is one example of a negative capacitance effect but you can think of it as a way of capturing way more charge than you normally would have.” Nirmaan Shanker, a graduate student in Salahuddin’s group, is also a co-lead author.
To scale up the energy storage capability of the films, the team needed to increase the film thickness without allowing it to relax out of the frustrated antiferroelectric-ferroelectric state. They found that by interspersing atomically thin layers of aluminum oxide after every few layers of HfO2-ZrO2, they could grow the films up to 100 nm thick while still retaining the desired properties.
Finally, working with collaborators at the MIT Lincoln Laboratory, the researchers integrated the films into three-dimensional microcapacitor structures, growing the precisely layered films in deep trenches cut into silicon with aspect ratios up to 100:1. These 3D trench capacitor structures are used in today’s DRAM capacitors and can achieve much higher capacitance per unit footprint compared to planar capacitors, allowing greater miniaturization and design flexibility. The properties of the resulting devices are record breaking: compared to the best electrostatic capacitors today, these microcapacitors have nine-times higher energy density and 170-times higher power density (80 mJ-cm-2 and 300 kW-cm-2, respectively).
“The energy and power density we got are much higher than we expected,” Salahuddin noted. “We’ve been developing negative capacitance materials for many years, but these results were quite surprising.”
These high-performance microcapacitors could help meet the growing demand for efficient, miniaturized energy storage in microdevices such as Internet-of-Things sensors, edge computing systems, and artificial intelligence processors. The researchers are now working on scaling up the technology and integrating it into full-size microchips, as well as pushing the fundamental materials science forward to improve the negative capacitance of these films even more.
Cheema added, “With this technology, we can finally start to realize energy storage and power delivery seamlessly integrated on-chip in very small sizes. It can open up a new realm of energy technologies for microelectronics.”
Parts of this work were performed at the Molecular Foundry, a DOE Office of Science nanoscience user facility located at Berkeley Lab.
**
This is quite interesting as a development for more miniaturization. The idea that a chip could substitute for an entire circuit board is almost a revolution all by itself. One wonders would there be anything to see other than the connection facilities on the chip? There might only be a connection and a heat sink to be seen.
The technology will very likely see market uptake. For now, there are rows of folks soldering up circuit boards in assembly line fashion by the millions.
Which means this tech will likely be more better and cheaper coming to consumers soon. Then off to a landfill someday with much less toxic elements.